Chapter 1 The Hypothalamo-Pituitary-Ovarian Axis
Understanding the iner-relationship between the different endocrine glands is important in practicing as a Reproductive Endocrinologist. No endocrine gland acts in isolation without affecting, or being affected by the other glands. In this field the pituitary, ovaries, thyroid and adrenal glands have special integrated roles in controlling our wellbeing and reproduction. Other glands are also important, but are outside the remit of this book. Each gland acts a subordinate to a central hypothalamic-pituitary co-ordinator. It is now evident that this central control which was once thought to be the maestro, is in fact continuously affected by its subordinate target gland. Nevertheless, the pituitary gland still remains an important part of the
neuroendocrine system through which the brain controls growth,
metabolism, general health and reproduction, among many other vital human functions. This
is affected through different neurotramitters which are conveyed first to the
hypothalamic nuclei which in turn control the pituitary gland. The
hypothalamo-pituitary axis is made of two developmentally different parts
called the neurohypophysis and adenohypophysis.
The
neurohypophysis
The neurohypophysis is developed from the ectoderm of
the diencephalon (mid brain). It is made of 3 different parts:
- The neural
lobe (the infundibular process)
- The median
eminence (the Infundibulum)
- Infundibular
stem
The neural lobe is connected to the median eminence
by the infundibular stem. It is made of nerve axons arising in the
hypothalamus with their final terminals ending in the vicinity of small
blood vessels. This neurovascular association lacks a blood brain barrier, and
is drained by the posterior hypophyseal veins. The cell bodies of these nerves
are located in the supraoptic and paraventricular nuclei. Accordingly, the neurohypophysis is not a true endocrine gland, and forms the terminal
stop for the release of oxytocin and antidiuretic hormone. These two hormones pass down as
granules in the nerve axons along the infundibular stem. The target organs for
oxytocin are the breasts and uterus, where as antidiuretic hormone exerts its
main effect though the collecting tubules of the kidneys. A detailed
description of the neurohypophysis structure and function is beyond the remit
of this chapter, which is mainly concerned with the development, maturation,
inter-relationships and aging of the hypothalamo-pituitary-ovarian axis. All respectable physiology books give detailed and illustrated manuscripts, and can be consulted when necessary.
The adenohypophysis
The adenohypophysis originates during the 4th
week of fetal life from the ectoderm of Rathke’s pouch which is a diverticulum
of the primitive foregut. It elongates cranially and contacts the neural
diverticulum destined to form the neurohypophysis
by the 5th week. It loses its connection to the foregut by the 6th
week. The adenohypophysis is fully developed by the 16th week of
intrauterine fetal life. By then, it is tightly close to the neurohypophysis, but
remains functionally different. It is also made of 3 different parts:
- The pars
distalis
- The pars
intermedia
- The pars
tuberalis
The pars distalis forms most the gland. It is
responsible for the secretion of follicle stimulating hormone (FSH), luteinising
hormone (LH), thyroid stimulating hormone (TSH), adrenocorticotrophic hormone (ACTH), growth
hormone (GH), prolactin, melanocyte stimulating hormone (MSH) and endorphins. Each of these hormones secretion is controlled through both neural and humoral
mechanisms. A negative feedback mechanism, affected at
the level of the pituitary gland and hypothalamus, coordinate the secretion of the target endocrine glands. It also prevents overproduction of the related tropic hormones by the pituitary gland. Neural control of the pars
distalis hormones is affected through neurotransmitters, which facilitate the
production of these tropic hormones. Prolactin stands out as the only pituitary hormone inhibited
through dopamine secretion.
The pars intermedia lies between the pars distalis
and infundibular process, hence its name. It is non-functional in adults and is
only seen during fetal life and in pregnant women. It is normally separated
from the pars distalis by the hypophyseal cleft. The pars tuberalis covers the
pituitary stalk as a collar and carries the portal vessels connecting the pars
distalis with the hypothalamus. Its exact endocrine function has not been conclusively
established, but was thought to have a role in the short loop feedback control
mechanism of gonadotrophins secretion.
In the context of this chapter, the main script will concentrate on the hypothalamo-pituitary-ovarian axis which is the main
neuroendocrine system responsible for the development of secondary female
sexual characteristics and reproduction. The adrenal and thyroid glands will be discussed in more detail separately in different chapters.
Development of the ovaries
Development of an adult female follows the following
steps:
- Chromosomal
sex
- Gonadal sex
- Genital sex
- Sex of
rearing.
Any malfunctioning at any point along this trail would
lead to physical and psychological abnormalities which could affect the sexual
identity, reproductive capacity and quality of life of the individual concerned.
However chromosomal sex is the most important and driving force behind this
sequence of developmental stages, depending whether a sperm with a Y or X
chromosome fertilises the egg. This would dictate the development of the
primitive gonad into a testicle or ovary respectively which in turn controls
the development of the genital organs. Testicular production of testosterone
and dihydrotestosterone would promote differentiation of internal as well as external
male genital organs. Failure of testicular development, rather than ovarian
development, would allow female external genitals differentiation from the
urogenital sinus. According to genital sex assignment at birth, an individual
would be brought up as a boy or a girl by the parents and society, which moulds
his or her identity through sex of rearing. However, the issue of gender
identity is a complex one, especially in women exposed to excessive amount of
androgens during the intrauterine period of life as in cases of congenital
adrenal hyperplasia.
The initial step in primitive gonadal development
entails genital ridge formation by thickening of the coelomic epithelium on the
medial aspect of the mesonephros. This is followed by migration of primordial germ
cells from the wall of the yolk sac to the genital ridge before and during
differentiation of the gonad into a testicle or an ovary. This is an
autosomally controlled process at this early stage which is similar in both
sexes. Further development of the primordial germ cells into the sex cords
stage depends on the presence of one X chromosome. However, further development
of the primitive gonads depends on the presence or absence of testicular
determining factor encoded in the sex determining region gene (SRY). This is located
in the short arm of the Y chromosome (Sinclair AH
1990 (1) and converts the
primitive gonads into testicles. Absence of this factor would allow growth of the
primitive gonads into ovaries instead. Further development beyond the primary
oocytes stage depends on the presence of two X chromosomes. In the absence of a
second X chromosome, the primordial follicles would undergo rapid atresia
leading to degeneration of the ovaries into streak gonads. The primitive gonad
would normally be destined to develop as an ovary by the 7th or 8th
week of intrauterine fetal life.
Maturation
of the HPO axis
Four different
stages have been recognised leading to full maturation of the HPO axis to its
adult state.
- Developmental
stage
- Inhibitory
stage
- Pubertal
stage
- adult stage
The first or developmental
stage begins with the differentiation of the different parts of the axis
during fetal life as described before. The system becomes functional during the
second trimester with maximum secretion of gonadotrophins by the pituitary
gland under the control of the fetal hypothalamus. This coincides with a
maximum number of 7 million primordial follicles in the ovaries by the 24th
weeks of intrauterine life. The negative feedback mechanism controlling
gonadotrophins releasing hormone (GNRH) secretion starts late during pregnancy
and is marked by progressive decline in gonadotrophins secretion by the fetal
pituitary gland. This phase is coupled by increased oocytes atresia and loss of
ovarian primordial follicles leaving behind only one million in both ovaries at
birth. Following birth and loss of the inhibitory placental steroids, the
neonatal hypothalamo-pituitary unit is reactivated resulting in more
gonadotrophins secretion which could exceed adult levels for about 3-4 months.
This again is followed by a slow decline in gonadotrophins secretion to almost
undetectable levels by the end of the second year of life.
This is followed by an inhibitory stage which extends up to the age of 8-9 years. This
juvenile hypothalamic pause is characterised by minimal, if any, production of GnRH.
The exact molecular changes which lead to this arrest of the hypothalamic GnRH
pulse generator are not fully explored. Central neural suppression is frequently
quoted as the main cause. However, there is substantial evidence now relating
such arrest to an inhibitory gamma aminobutyric acid (GABA) effect on the
hypothalamus. There is increased level of the hypothalamic mRNAs encoding for
the enzyme glutamic acid decarboxylase, which is responsible for the production
of GABA, at the time of GnRH generator arrest (El
Majdoubi et al 2000 (2) and El Majdoubi et al 2000 (3)). Reduction of
this inhibitory tone is associated with the onset of puberty (Mitsushima et al 1994 (4); Terasawa and Fernandez 2001 (5).
A similar effect has also been related
to a high level of melatonin during the inhibitory period of development (Garcia et al 2002 (6). A reduction in melatonin level
to a critical value by the age of 10 years is associated with a release of this
inhibitory effect, and increased production of GnRH and LH (Aleandri et al 1996 (7). Removing the ovaries during
this stage would not lead to any increase in GnRH or gonadotrophins secretion, which
is a reflection of the extent of such hypothalamic pause. The pituitary gland
itself is less responsive to intravenous infusion or subcutaneous
administration of GnRH during this inhibitory stage of life. This is a
reflection of the fact that some aspects of the pituitary gland maturation might
be independent of the hypothalamic GnRH pulse generation.
The pubertal
stage would follow, and it signals the start of the HPO axis maturation. It
is an age dependent process which follows genetically controlled CNS maturation
necessary for the pulsatile release of GnRH (Ojeda 2006
(8). For some time, this process was thought to be related to adrenarche
which indicates the start of adrenal androgens secretion, by the zona
reticularis. There is gradual increase in adrenal androgen secretion over a
period of 2 years before the onset of puberty. This is coupled with a
progressive increase in the size of the zona reticularis of the adrenal cortex.
However, this concept is no longer valid and other factors are becoming more
evident as initiators or facilitators of puberty onset. Attaining a minimum BMI with minimum and
critical body fat mass of about 22% is one such factor. This results in an
increase in the level of blood leptin which is a peptide hormone produced by
adipocytes. At a certain blood level threshold, leptin would facilitate the
development of puberty, so long as other critical control mechanisms are
operational (Mann and Plant 2002 (9).
Developmentally, leptin reflects the amount of body fat and energy reserve. It signals
to the brain that enough energy reserves are available for initiating
reproductive function. Its level starts rising by the age of 7 - 8 years and
peaks by the age of 13 – 15 years. Thereafter, the levels of serum leptin would
parallel those of LH and oestradiol. Leptin has been found to induce
gonadotrophins production by stimulating GnRH pulse generation. It also
increases LH more than FSH by acting directly at the level of the pituitary
gland. Another facilitatory factor for initiation
of puberty is the kissprotein, which is encoded by the KiSS-1 gene, and its
receptor GPR54. This KiSS-1/GPR54 system is an important regulator of puberty
in all mammals. KiSS-1 mRNA and GPR54 mRNA are
both increased in the hypothalamus at the onset of puberty, with robust expression
in the region of the arcuate nucleus (Shahab et al 2005
(10).
Other important endocrine changes during early
puberty include increase in the levels of growth hormone releasing factor and
growth hormone (GH) itself, mainly at night time. GH has been shown to
stimulate FSH induced granulosa cell differentiation. It also increases
intraovarian levels of IGF-1, and enhance ovarian response to gonadotrophins.
Together with IGF-1, they exert a paracrine intraovarian control on
steroidogensis.
Changes in the level of leptin and KiSS-1/GPR54 mRNA
transduction are coupled by a reduction in the hypothalamic GABA tone as
already mentioned to coincide with the onset of puberty. All these factors,
plus a genetically controlled CNS maturation lead to a release of the block on
the GnRH pulse generation, allowing the appearance of large nocturnal GnRH
pulses and nocturnal LH secretion, during sleep.
With final maturation of the system into the fourth
or adult stage, these large nocturnal
LH pulses become more frequent with smaller amplitude, probably secondary to
increased nocturnal dopamine activity. A 24-hour pulsatile GnRH and LH
secretion pattern forms the next step in this adult maturation stage, which is
later on capped by maturation of the oestrogen positive feedback and LH surge
mechanisms.
GnRH and gonadotrophins
GnRH is a
decapeptide (10 amino acids) produced in pulses by the median eminence of the
hypothalamus, which do not necessarily correspond to follicle stimulating
hormone (FSH) or luteinising hormone (LH) pulses. Development of the positive
feedback mechanism indicates the final step in the maturation of the HPO axis.
It might take a couple of years to develop after menarche and is one of the
reasons why the early menstrual cycles are not ovulatory. It is also the first mechanism
to be lost following any hypothalamic GnRH dysfunction. With this maturation
step, very small GnRH pulses upregulate their own receptors in the pituitary
gland without causing any LH secretion. This pattern is seen just before an LH
surge which is affected by a dose and time controlled exposure of the pituitary
gland to oestradiol just before ovulation. For organised follicular development,
FSH and LH should be produced in tonic and cyclic patterns at different parts
of the cycle. Tonic release indicates continuous production of both hormones
and the cyclic production is responsible for the positive feedback mechanism.
Both tonic and cyclic production are pulsatile in nature. The short first half
life of LH (30 minutes) allows better perception of the pulsatile nature of its
production than FSH which has a longer first half life of 60 minutes. This
difference is secondary to the amount of carbohydrate moiety in the two
hormones, being higher in FSH. Such differences in half life could explain the
lack of synchrony between the pulse patterns of both hormones in relation to the
short half life of GnRH pulses, which is 2.7 minutes. Furthermore, some GnRH
pulses might not stimulate LH production due to temporary refractoriness of the
pituitary gland. One important extra observation was that GnRH in the
peripheral blood could be produced by the pancreas, or could leak from the
organum vasculosum, which is outside the blood brain barrier.
Sustained large GnRH pulses could desensitise the pituitary
gland by downregulating its own receptors on the surface of the gonadotrophs. This
suppression is always preceded by a short flare period with increased blood
levels of gonadotrophins and oestradiol. GnRH receptors are also found within
the ovaries at the follicular level. Accordingly, sustained non-physiological
doses of GnRH could interfere directly with ovarian function. They could reduce
FSH induction of follicular aromatase enzymatic activity, leading to reduced
oestradiol production. Furthermore, downregulation of ovarian LH receptors
would also result in reduced progesterone production.
Both FSH and LH are glycoproteins with identical a chain but have
different amino acid sequence in their β chains. FSH receptors are located in
the granulosa cells where as LH has receptors in both granulosa and theca
cells. As for other protein hormones, these receptors are located in the cell
membrane and have short half life of 30 minutes, indicating rapid turnover. Only
2% of the receptors need to be occupied to initiate a local response. Follicles
are responsive to FSH stimulation only when they reach or exceed 60-cell stage.
The characteristic functions of FSH could be summarised as follows:
- It
stimulates granulosa cells hyperplasia
- It stimulates
accumulation of the liquor folliculi during development of the antral
follicles
- It increases
its own receptors as well as LH receptors
- It induces
the aromatase enzyme activity for the conversion of androgens to
oestrogen.
- It initiates
the cumulus expansion and separation of the oocytes and cumulus mass from
the rest of the granulosa cells
before ovulation
- FSH surge
secures enough LH receptors to allow adequate luteinization after the LH
surge.
On the other
hand, LH has got the following characteristics - It stimulates
steroidogensis and production of progesterone and androgens by the theca cells
- LH surge is
responsible for the actual act of ovulation. This is dependent on the pituitary
gland LH reserve, level of oestradiol attained, and the duration of exposure of
the hypothalamus to this high oestrogen level.
- Luteinization of
the granulosa cells necessary for the production of progesterone.
- It also
stimulates resumption of meiosis with extrusion of the first polar body just
before ovulation.
The LH surge
This is a very
intricate process, and could easily be affected adversely leading to abnormal
ovulation or even anovulation. Different events occur at the level of the
hypothalamus, pituitary gland, and ovary in preparation for this event.
· The hypothalamus
secrets small and rapid GnRH pulses to upregulate its own receptors at the
level of the pituitary gland.
· This increase in
pituitary GnRH receptors level is also associated with an increase in the
pituitary storage of LH itself. The LH surge occurs when both attain a critical
level, and the pituitary gland is exposed to a critical level of oestradiol for
a critical period of time.
· Changes within
the ovary include increased production of oestradiol before the LH surge,
neovascularisation, increased levels of prostaglandins and plasmin. These are
also associated with increased osmotic pressure and fluid influx into the
follicle.
The actual LH surge
starts at midnight. It peaks just before noon on the following day and lasts
for about 48 hours, when the accumulated pituitary LH reserve is exhausted.
Ovulation is expected to occur within 36-40 hours after the start of the LH surge.
The negative feedback
For completion purpose,
factors controlling the gonadotrophins negative feedback should be alluded to.
Both oestradiol and progesterone could have a negative effect at the level of
the hypothalamus. Increased level of oestrogen is associated with increased
hypothalamic dopamines and reduced adrenergic activity. Progesterone also
increases the level of hypothalamic endorphins; hence both steroids could
affect GnRH pulse generation. At the level of the pituitary gland both
oestrogen and progesterone could reduce gonadotrophins secretion by affecting
GnRH postreceptor activity, but not the receptors themselves.
Factors affecting the HPO axis
Many factors
could affect the HPO axis function with variable consequences on the ovaries.
The initial response would be loss of the LH surge, with further progression to
inadequate ovulation, dysfunctional uterine bleeding, infrequent ovulation and finally
anovulation. These factors could be environmental, neural or endocrine in
nature. The vulnerability of the HPO axis is reflected by the fact that it is modulated
by corticotrophins, cortisol, adrenaline, nor adrenaline dopamine, serotonin,
acetylcholine and gamma amino butyric acid, just as examples.
The most
important environmental factors which could affect the hypothalamo-pituitary
axis are excessive weight gain or loss, bulimia even without weight change,
excessive exercise, morbid stress, depression and recreational drugs. They could
affect the hypothalamus in different ways and hence interfere with GnRH pulse
generation. They would be discussed in more detail in different chapters in
this book.
The
interrelationship between the different endocrine glands makes it difficult, if
not impossible, for a gynaecologist to practise reproductive medicine without
thorough knowledge of these interactions. This is especially so for dysfunctions
of the thyroid and adrenal glands as well as hyperprolactinaemia. More
information about these inter-relations would be given in Chapters 3 and 4. Polycystic
ovarian syndrome is the most common female endocrine dysfunction and would be
discussed in chapter 6 in this book. Different LH and FSH pulse patterns have
been described (Abdel-Gadir et al (11), but
there is general agreement regarding increased LH pulse amplitude and probably
pulse frequency as well. However, the main characteristics of the syndrome are hyperandrogenisation
and anovulation.
Drugs both
prescribed and recreational could affect the HPO axis. The most commonly
prescribed ones include antipsychotic and certain anti-hypertensive drugs. On the other hand, alcohol forms the most
commonly used recreational chemical or drug. It has been shown to be detrimental
on pubertal development, disrupts normal menstrual function and affects
postmenopausal hormone levels (Emanuele et al 2002 (12).
The effect of alcohol on puberty involves both the HPO axis, growth
hormone and insulin growth factor-1 activities, which are functionally
interrelated. Alcohol consumption among adolescent girls between the ages of
12-18 years was shown to suppress oestrogen levels for as long as 2 weeks, even
after moderate consumption (Block et al 1993 (13).
Lower LH (Rettori et al 1987 (14), and growth
hormone (Dees and Skelley 1990 (15) blood levels
have been reported after alcohol consumption. Furthermore, the development of
regular menstrual pattern after menarche was similarly affected by alcohol intake
(Dees et al 2000 (16).
The effect of
alcohol on oestradiol level after the menopause depended on the amount taken
and whether that individual was on HRT or not. Acute alcohol exposure has been
shown to cause temporary increase in oestradiol level in women taking HRT. This
was thought to follow decreased conversion of oestradiol to oestrone (Purohit 2000 (17). There was no similar effect on
women not using HRT. An opposite effect of chronic or high alcohol consumption
was noticed on the level of oestradiol in women using HRT (Johannes et al 1997 (18). The effects of many other
recreational drugs including marijuana and cocaine have also been examined both
in the acute phase and after chronic use.
Ageing of the HPO axis
The reproductive episode in human beings is very short.
Maximum fertility potential spans for only few years between the ages of 23-29
years. This episode is preceded and followed by times of reduced fertility potential
due to immaturity, to start with, and aging thereafter of the HPO axis respectively.
To be more accurate, aging of the HPO axis starts during fetal life after the
24th week of pregnancy. Accelerated loss of the primary follicles
starts at that time, and drops from 7 million to one million at birth as
mentioned before. This is followed by further atresia and only 0.7 million
would be found in both ovaries by the time of menarche. It is estimated that
about 100 follicles would be found by the time of menopause. Such ovarian aging
is also associated with increased oocytes chromosomal abnormalities after the
age of 35 years. It is estimated that 1: 4 eggs would be aneuploid by the age
of 35, 1: 2 by 42 years and >80% of the eggs would be similarly abnormal by
the age of 44 years (R). Accordingly, ovarian
aging is marked with the presence of fewer oocytes with higher chromosomal
abnormalities. The major and most significant early endocrine change during the same
period is a substantial fall in the circulating levels of inhibin-B, with no
significant change in inhibin-A or oestradiol. This would later progress on to a
decline in inhibin-A and oestradiol blood levels, and a rise in FSH level
without any further change in inhibin-B (Burger et al
2002 (19)
At the same time
an independent hypothalamic aging process has been documented, not related to
the ovaries. This was thought to be the cause of the high FSH blood levels and
changes in LH pulse pattern during the early follicular phase of regularly
menstruating women in their late thirties, compared to younger women. Further
arguments have also been put forward to prove this concept of hypothalamic
aging including: - The occurrence of
hot flushes in women between the ages of 35-40 years despite having regular
menstrual cycles
- The age dependent
changes in FSH level could be explained by changes in the negative feedback
affected by inhibin B and oestradiol in only a fraction of women.
Unfortunately,
FSH is not a very reliable marker of ovarian ageing, at least in its early
stages. This is because of the following reasons:
1. It is produced in
pulses and timing the blood sample within that pulse could affect the FSH
level. The peak, the rough or any point in between could be represented.
2. High FSH could be
seen during some but not all cycles during the early stages of the climacteric
period. This pattern becomes more frequent with time, till it becomes established
during all cycles.
3. High oestradiol
in the early follicular phase could suppress FSH production and lead to a low
or normal FSH blood level. Accordingly, oestradiol level should be tested in
the same sample for endocrine coupling. A high level ≥200 pmol/l is equally
important as a high FSH level in reflecting a reduced ovarian reserve. Transvaginal
ultrasound examination on day 2 or 3 of the cycle might show:
a. Rapid follicular recruitment
with a single advanced or large follicle. Such follicle could produce high
oestrogen (>200 pmol/l) which would affect FSH level. In such cases rapid
growth and maturation of the dominant follicle would lead to a short follicular
phase. This explains why polymenorrhoea is the first sign of the climacteric or
incipient ovarian failure.
b. Multiple follicles
recruitment, with many of them growing to a medium size during the early part
of the follicular phase, could lead to high oestradiol level and falsely normal
FSH blood level.
Figure 1 shows 18.5 mm follicle in the left
ovary on day 5 of the cycle which was diagnostic of rapid follicular
recruitment. This patient had polymenorrhoea with short follicular phase.
Note the lack of any activity in the right ovary. Figure 2 demonstrates
multiple follicular recruitment on the 3rd day of the cycle. The patient was
31 years old. She had one 12 mm follicle in the left ovary and 3 others in
the right one measuring 14.5 mm, 12.5 mm and 10.5 mm respectively. She
also had short menstrual cycles. Note the small size of the left ovary. Her
FSH blood level was 6.8 IU/L and oestradiol 248 pmol/l on the same day. More information about other conditions which may affect FSH blood level can be found in Chapter 14
Normally, a
single dominant follicle would produce enough oestradiol to switch off FSH
production causing demise of the other recruited ones. However, during the late
30s or early 40s one follicle would not produce enough oestradiol to switch off
FSH production by the pituitary gland, during the mid follicular phase. More
than one follicle would then be needed to produce that amount of oestradiol.
Accordingly, two or more follicles would reach maturation and ovulate at the
same time probably with a wide ovulation window, depending on their size. This
explains why binovular twins are more common in women in their late 30s, than
in younger ones. Accordingly, such twining is a sign of reduced HPO axis
integrity with compromised negative feedback mechanism to oestradiol. It is
definitely not a sign of increased or enhanced fertility potential, as commonly
thought. 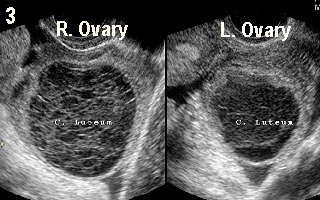 | 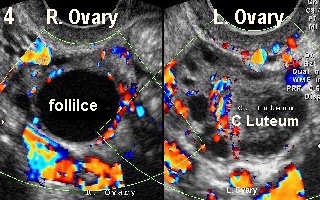 |
Figure 3 shows double ovulation in a spontaneous monitored cycle with a
corpus luteum in each ovary, in a 37 year old woman. On the other hand, Figure 4 shows a dominant follicle in the right ovary and a corpus luteum in
the left one. A colour Doppler copy of this picture on the back cover shows a
good vascular rim around the dominant follicle in the right ovary indicating
imminent ovulation in a different patient of almost similar age. This case
demonstrated a wide ovulation window with two follicles ovulating at different
times, which is not uncommon during natural cycles in women in their late 30s
It
can be safely stated that a single normal FSH test has a very low diagnostic
or even screening value for ovarian reserve according to the facts mentioned
before. On the other hand a single high level >13.0 IU/L performed in a
reputable laboratory would indicate reduced ovarian reserve, even if repeating
the test in more than one occasion showed normal values (Scott et al (20) and Martin et al (21). Accordingly, such
repetition in subsequent cycles in a woman who had a single high value is not indicated
and adds unnecessary costs.
Different
ways have been tried to improve the predictive value of FSH as a predictor of
ovarian reserve including: - The clomid challenge test has been used
extensively for that purpose.
- Following a basal blood sample on day 3 of the
cycle, the patient is asked to take 100 mg of clomid for 5 days.
- By day 10 of
the cycle, the level of FSH is again assayed.
- Women with incipient ovarian
failure would have blood FSH level ≥13.0 IU/L.
- Normally, FSH level should be
lower due to the negative effect of oestradiol and inhibin B produced by the
follicle at this stage of the cycle.
These
tests have been used as an indirect measurement of the ovarian reserve or
follicular pool, and to predict the clinical response to ovulation induction in
women >35 years of age, who are seeking to get pregnant. They are not sensitive,
and better tests are now available for that purpose. They are not necessary
outside this context and could not be used to predict future fertility or the exact time of
cessation of fertility (Maseelall and McGovern 2008 (22), or the age at menopause. Inhibin B blood
levels and antral follicle count on day 3 of the cycle have been used for some
time, but have been superseded by antimullerian hormone (Knauff EA et al, 2009 (23), which has the added
value of having a non-variable level at different times of the cycle.
Accordingly, the test could be conducted at any time, and not restricted to the
early follicular phase. It is especially useful for the assessment of
follicular pool in young women with relatively high FSH and regular periods
(incipient ovarian failure) or high FSH and oligomenorrhoea (transitional
ovarian failure).
Other endocrine changes which
occur with aging, but are not related to the climacteric are progressive
decline in the level of dehydroepiandrosterone and dehydroepiandrosterone sulphate.
On the other hand, despite a 50% fall in the level of testosterone between the
ages of 20-40 yeas, little if any changes occur during the transitional period
before the menopause, and its level might even rise thereafter (Burger et al 2002 (24).
Younger women might need to have
their ovarian reserve tested after a shorter period of infertility in the
following circumstances: - Following ovarian surgery
- Following ovarian irradiation
- Women with a single ovary
- Irregular periods with family history of early menopause
- Associated autoimmune problems mainly of thyroid and adrenal nature
Summary
It is
evident that the function and integrity of the HPO axis as a single unit can be affected by many factors, from within or without the axis itself. Accordingly,
knowledge of the pathophysiology of this system relies on thorough knowledge of
the normal and abnormal function of the other endocrine glands, which may interact to disrupt its function. This knowledge will hopefully be provided in
the different chapters included in this book. In essences, this chapter was meant
to be a platform or a launching pad for the rest of the book.
References
1. Sinclair AH, Berta P, Palmer MS, et al. A
gene from the human sex-determining region encodes a protein with homology to a
conserved DNA-binding motif. Nature 1990; 346:240-244.
2. El Majdoubi M, Sahu A and Plant TM. Changes
in hypothalamic gene expression associated with the arrest of pulsatile
gonadotrophin-releasing hormone release during infancy in the agonadal male
rhesus monkey (Macaca mulatto). Endocrinology 2000; 141: 3273-3277.
3. El Majdoubi M, Sahu A, Ramaswamy S and Plant
TM. Neuropeptide Y: a hypothalamic break restraining the onset of puberty in
primates. Proc Natl Acad Sci USA 2000, 97: 6179-6184.
4. Mitsushima D, Hei D and Terasawa E. Alpha
aminobutyric acid is an inhibitory neurotransmitter restricting the release of
luteinising hormone-releasing hormone before onset of puberty. Proc Natl Acad
Sci USA 1994; 91; 395-399.
5. Terasawa E, Fernandez DL. Neurobiological
mechanisms of the onset of puberty in primates. Endo Rev 2001; 22: 111-151.
6. Murcia García J, Muňoz Hoyos A, Molina
Carballo A, Fernández García JM, Narbona López E and Uberos Fernández J.
Puberty and melatonin. An Esp Pediatr 2002; 57(2): 121 - 126.
7. Aleandri V, Spina V and Morini A. The pineal
gland and reproduction. Hum Reprod Update 1996; 2(3): 225 - 235.
8. Ojda SR, Roth C, Mungenast A, Heger S, Mastronard C, Parent AS, Lomniczi A, Jung H. Neuroendocrine mechanisms
controlling female puberty: new approaches, new concepts; Int J Androl. 2006; 29(1): 256 -263.
9.
Mann DR and Plant TM. Leptin and pubertal
development. Semin Reprod Med 2002; 20(2): 93-102.
10.Shahab M, Mastronardi C,
Seminara SB, Crowley WF and Ojeda SR. Increased hypothalamic GPR54 signalling:
a potential mechanism for initiation of puberty in primates. PNAS 2005; 1026):
2129 - 2134
11.Abdel Gadir A, Khatim
MS, Mowafi RS, Alnaser MI, Muharib NS and Shaw RW. Implications of
ultrasonically diagnosed polycystic ovaries. II. Studies of dynamic and
pulsatile hormonal patterns. 1992; 7(4): 458 - 461.
12.Emanuele MA, Wezeman F
and Emanuele N. Alcohol’s effects on female reproductive function. Alcohol Res
Health 2002, 26(4): 274 - 281.
13.Block GD, Yamamoto ME,
Mallick A and Styche AJ. Effects on pubertal hormone by ethanol abuse in
adolescents. Alcoholism: Clinical and Experimental Research 1993; 17: 505
14.Rettori V, Skelley CW,
McCann SM and Dees WL. Detrimental effect of short-term ethanol exposure on reproductive
function in the female rat. Biology of Reproduction 1987; 37: 1089 - 1096.
15.Dees WL and Skelley CW.
Effects of ethanol during the onset of female puberty. Neuroendocrinology 1990;
51: 64-9.
16.Dees WL, Dissen GA,
Hiney JK et al. Alcohol ingestion inhibits the increased secretion of
puberty-related hormones in the developing female Rhesus monkey. Endocrinology
2000; 141: 1325-31.
17.Purohit V. Can alcohol
promote aromatization of androgens to oestrogens? A review. Alcohol 2000; 22:
123 - 127.
18.Johannes C. Crawford S
and McKinley S. The effect of alcohol and oestrogen replacement therapy (HRT)
on oestrogen levels in postmenopausal women. A J Epidemiol 1997; 145: S1.
19. Burger HG, Cahir N, Roberston DM, Groome NP, Dudley E, Green A, Dennerstein L. Serum inhibin A and B fall
differently as FSH rises in perimenopausal women. Clin Endocrinol (Oxf) 1998;
48(6): 809 - 813.
20.Scott RT Jr,
Hofmann GE, Oehninger S, Muasher SJ. Intercycle variability of day 3
follicle-stimulating hormone level and its effect on stimulation quality in
vitro fertilization. Fertil Steril 1990; 54: 297 – 302.
21.Martin JS,
Nisker JA, Tummon IS, Daniel SA, Auckland JL, Feyles V. Future in vitro
fertilization pregnancy potential of women with variably elevated day 3
follicle-stimulating hormone levels. Fertil Steril 1996; 65: 1238 – 1240.
22.Maseelall PB, McGovern PG. Ovarian reserve screening: what
the general gynaecologist should know. Women Health 2008; 4(3): 291 -3 00.
23. Knauff EA, Eijkemans MJ, Lambalk CB, ten Kate-Booiji MJ, Hoek A, Beerendonk CC, Laven JS, Goverde AJ, Broekmans FJ, et al. Anti-Mullerian hormone, inhibin B and antral follicle count in young women with
ovarian failure. J Clin Endocrinol Metab 2009; 94(3): 786 - 792.
24.Burger HG, Dudley EC, Robertson DM and Dennerstein L. Hormonal changes in the
menopause transition. Recent Prog Horm Res 2002; 57: 257 - 275.
|